
For example, genetic variation can change how people are affected by drinking coffee. One person might drink a lot of coffee without feeling the effects, while another person cannot handle more than a cup. In this case, one person’s genetic variant of the enzyme that metabolizes caffeine is more efficient, while another variant is less efficient in the other person. Your ability to metabolize caffeine is dependent on which variant you carry.
Similarly, some people are less efficient at metabolizing environmental chemicals, putting them at a higher risk for disease. While these variants can create a genetic susceptibility to disease, the environmental exposure is still required for the disease to manifest.1
Some gene variants can be beneficial, providing protection against the harmful effects of outside agents. That said, the risks for common chronic diseases, such as cancer, Parkinson’s disease, and diabetes, result from the complex interactions between environmental exposures and gene variants.2
Examples of Gene-Environment Interactions
Below are two gene-environment interactions that are known to increase the risk for specific disease outcomes.
Organophosphate pesticides & Parkinson’s disease
Sporadic (non-familial) Parkinson’s disease (PD) manifests from a combination of multiple gene variants, environmental exposures, and lifestyle choices. Organophosphate (OP) pesticides, commonly used in agriculture, are neurotoxicants that have been implicated in the development of PD.3
The PON1 gene encodes for the enzyme paraoxonase 1, which metabolizes organophosphates.4 Genetic variation in the PON1 gene leads some people to have genetic susceptibility to the toxic actions of OP pesticides. When exposed to OPs, individuals who carry a less efficient variant of PON1 have double the risk of developing Parkinson’s disease versus a person who has a more efficient version of the same enzyme.5
Air pollution & asthma
Two genes are known to be involved in detoxifying exposures to air pollution – the glutathione (GST) gene and the epoxide hydrolase (EPHX1) gene. Both genes have variants associated with an increased risk of developing asthma.
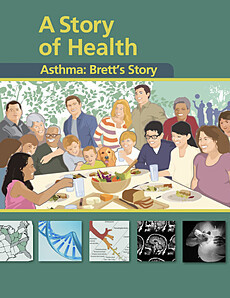
The risk of asthma increases 50% if an individual carries a poorly functioning EPHX1 variant. The risk increases four-fold if a person carries poorly functioning copies of both GST and EPHX1. Even more striking is the interaction between these variants and exposure to air pollution. Children who have both high-risk variants and live close to a major roadway have a nine-fold increased risk of developing asthma.6
Read more about this interaction and the environmental drivers of asthma in CHE's A Story of Health chapter on Asthma: Brett's Story.
Epigenetics
Whereas the genome is the full code for all of the proteins that make up a human being, the epigenome, in its simplest form, is a system of tags that surrounds the genome and controls what it does.
These tags turn genes on or off. It is through epigenetic mechanisms that cells become specialized.7 Your development from one fertilized egg into a collection of 200 cell types across 3 trillion cells occurs through epigenetic regulation.8 For example, genes that allow cells to detect light are turned on in the eye, but not the liver.
Humans need different genes to function at different times, depending on whether cells need to repair themselves, fight off intruders, divide into two cells, or function as part of an organ. Epigenetic mechanisms allow this to happen.9
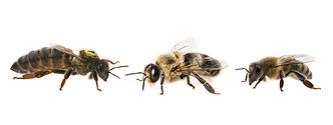
Bees illustrate an example of environmental exposure changing gene expression. A worker bee and a queen bee are genetically identical. When a developing bee is fed royal jelly, an epigenetic modification is made to the reproductive genes and they turn on. Only developing bees fed royal jelly can grow into queen bees. Only these bees can reproduce. The genes are not changed, but their expression has.
Occupational and environmental exposures can change gene expression through epigenetic mechanisms. In humans, hormones circulating in a mother’s bloodstream affect the developing reproductive system of the fetus through epigenetic changes. Endocrine disrupting chemicals (EDCs) can mimic or disrupt the action of hormones and disrupt this natural process. For example, EDCs can alter gene function in pathways associated with infertility, obesity, cancer, and osteoporosis.10
Lifelong impacts
The epigenome is heritable between generations of cells. Each time a cell divides, not only is the DNA copied, but the epigenome around that DNA must also be copied. This way, the daughter cell is as specialized as the cell it came from. It is especially important that the epigenome is copied correctly in cells of developing fetuses and children.
Folate is an important part of the epigenetic tagging process. If there is not enough folate to supply the dividing epigenome with the tags it needs, disorders such as spina bifida can result. This is one reason why doctors recommend folic acid supplements to pregnant women.11 Maternal nutrition is especially important to ensuring there is enough folate, as well as other precursors, available for the epigenetic replication processes to occur accurately.12
One active area of epigenetic research is in cancer. Alterations in the epigenome can change whether genes are on or off. Those changes can affect uncontrolled cell growth and immune responses.13 Additionally, there are specific genes in the genome that should never be turned on (such as retrotransposons). Epigenetic mechanisms are crucial in keeping those genes silenced. In several types of cancer, those genes are active.
Studies in animals show that when developing young are exposed to the EDC bisphenol A (BPA), these silenced genes can turn on and disrupt normal genomic functioning. For example, rodents exposed to BPA in utero have a greater risk of developing cancer, along with obesity and diabetes. Interestingly, when the BPA-exposed pregnant rodent was also given a folic acid supplement, those risks were reduced.14
Windows of vulnerability
During fetal development and infancy, the growing child’s body must learn how to function as a whole organism that is constantly adapting to specific environmental conditions. The epigenome is especially vulnerable to environmental exposures and toxicants during these developmental stages.
Because development is a period of rapid cell division, effects of epigenetic alteration can last a lifetime. Organ systems undergo developmental programming in utero, and this programming governs an individual's capacity to adapt to physical and metabolic stressors later in life. Establishing healthy epigenetic profiles across dividing cells is important for health in adulthood.
Transgenerational effects
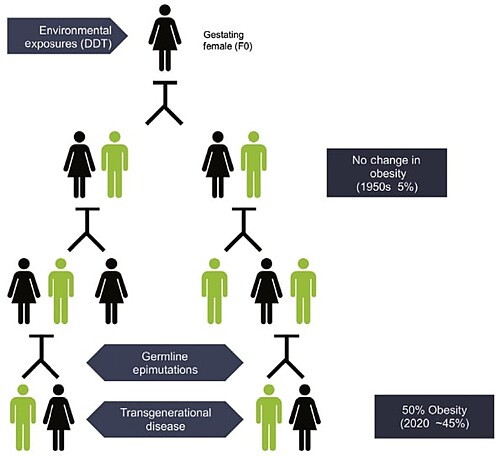
Chemical exposures can have transgenerational effects through epigenetics.
For example, when a person pregnant with a female fetus is exposed to EDCs, that represents three generations of exposures: The mother (F0), the fetus (F1), and the fetus’ already present germ cells and gametes (F2). If the 4th generation (F3) – that is, the great-grandchild of the exposed mother – also shows effects, then those effects are considered transgenerational. This represents health effects in individuals who were never personally exposed. Instead, their exposures were ancestral.
One study found that exposure to DDT could have transgenerational effects on the epigenome, increasing a person’s great-grandchildren’s chances of having obesity.16
Another example of epigenetic information being transferred between generations was demonstrated by rodent research on the endocrine disruptive fungicide vinclozolin. When pregnant female rats (F0 generation) were exposed to vinclozolin, the third generation (F3) experienced reproductive and kidney abnormalities. The researchers believe this was mediated by epigenetic markers.17
Exposures that Alter Epigenetic Function
Laboratory and human epidemiologic data demonstrate that common exposures have the ability to alter the epigenome in ways that can increase risk of chronic diseases, including cancer. The exposures described here can be found in everyday products such as motor vehicle exhaust, foods, household furniture, children’s products, and electronics.
There is strong evidence connecting the following exposures with the listed health outcomes via epigenetic effects. These exposures can also have other health effects that are not mediated through epigenetic mechanisms.
Air Pollution
|
Studies demonstrate that the components of air pollution can have these effects:
These effects can increase the risk for cardiopulmonary diseases, cancer, asthma, neurological diseases, and early aging. See our air pollution webpage.18 |
Endocrine Disrupting Chemicals (EDCs)
|
Many chemicals in this class can permanently change gene expression during the first trimester of pregnancy (organogenesis). They can also alter gene expression during development as a whole, alter hormonal signaling networks, and alter the expression of detoxifying enzymes. This can increase the risk for childhood chronic diseases, obesity, reproductive disorders, immune dysfunction, and cancer.19 See our EDCs webpage. |
Heavy Metals |
Heavy metals, including methylmercury, arsenic, cadmium, copper, iron, aluminum, and nickel, can disrupt epigenetic function. These exposures can change gene expression in the brain, activate transposable elements, alter the fetal epigenome, alter tumor suppression, alter gene expression, and alter neural growth expression. The resulting epigenetic alterations can increase the risk for poor birth outcomes, developmental abnormalities, cancer, and neurodegeneration.20 |
Persistent Organic Pollutants |
Many chemicals in this class can alter:
These changes increase the risk of cognitive developmental disabilities, reduced fetal growth, reproductive disorders, cancer, and autoimmune diseases.21 |
Stress |
Environments that are threatening, uncontrollable, or unpredictable can stimulate a stress response in the body, and chronic stress can erode a person's overall health.22 When experienced early in life, stressors can alter the epigenetic markers on a specific gene in the brain that affects the ability of the stress response system to turn off. Lab studies have shown that early-life stress, even in utero, can cause an epigenetic change in the brain that makes individuals more responsive to stressful stimuli. This heightened response increases the risk for behavior changes in adulthood, reduced parent-offspring interactions, and cardiovascular disease. Humans who have heightened stress responses are at a greater risk for anxiety, hypertension, obesity, type 2 diabetes, and autoimmune disorders. Human studies have shown that suicide completers also carry this epigenetic change in the brain. Though this research is in its infancy, it sheds light on the importance of early life experiences to lifelong health.23 |
Our expanding appreciation of the environment’s ability to affect genomic function through epigenetic changes argues for a precautionary approach to chemical and other hazardous exposures during development. We have an ethical responsibility to ensure that our children live in an environment in which they can reach and maintain their full potential, free of chemicals and other preventable exposures that cause adverse epigenetic changes.
This page was last revised in October 2024 by CHE’s Science Writer Matt Lilley, with input from Dr. Rachel Massey, and editing support from CHE Director Kristin Schafer.
CHE invites our partners to submit corrections and clarifications to this page. Please include links to research to support your submissions through the comment form on our Contact page.